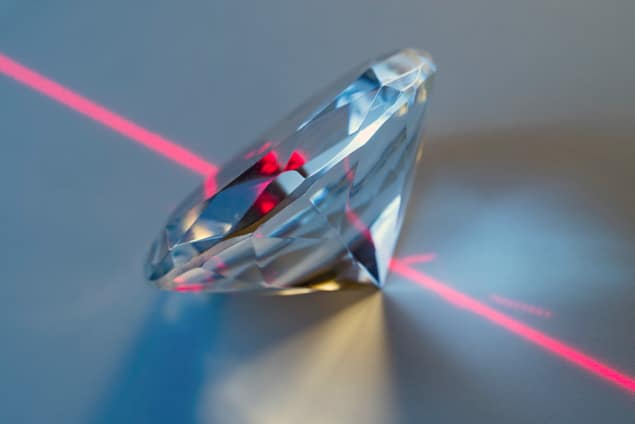
A handful of spins in diamond have shone new light on one of the most enduring mysteries in physics – how the objective reality of classical physics emerges from the murky, probabilistic quantum world. Physicists in Germany and the US have used nitrogen-vacancy (NV) centres in diamond to demonstrate “quantum Darwinism”, whereby the “fittest” states of a system survive and proliferate in the transition between the quantum and classical worlds.
In the past, physicists tended to view the classical and quantum worlds as being divided by an abrupt barrier that makes a fundamental distinction between the familiar macroscopic (classical) and the unfamiliar microscopic (quantum) realms. But in recent decades that view has changed. Many experts now think that the transition is gradual, and that the definite classical states we measure come from probabilistic quantum states progressively (although very quickly) losing their coherence as they become ever more entangled with their environment.
Quantum Darwinism, put forward by Wojciech Zurek of Los Alamos National Laboratory in New Mexico, argues that the classical states we perceive are robust quantum states that can survive entanglement during decoherence. His theoretical framework posits that the information about these states will be duplicated many times and disseminated throughout the environment. Just as natural selection tells us that the fittest individuals in a species must survive to reproduce in great numbers and so go on to shape evolution, the fittest quantum states will be copied and appear classical. This redundancy means that many individual observers will measure any given state as having the same value, so ensuring objective reality.
Isolated spins
To try and observe the redundancy experimentally, Fedor Jelezko of Ulm University in Germany and other experimentalists joined up with Zurek and some theorist colleagues. The team focussed on NV centres, which occur when two adjacent carbon atoms within a diamond lattice are replaced with a nitrogen atom and an empty lattice site. The nitrogen atom has an extra electron that remains unpaired. This behaves as an isolated spin – which can be up, down or in a superposition of the two. The spin state can be probed in a well-established process that involves illuminating the diamond with laser light and recording the florescence given off.
The researchers set out to monitor how the NV spin interacts with the spins of several neighbouring carbon atoms. Most carbon in the diamond is carbon-12, which has zero spin. However, around 1% of the atoms are carbon-13, which has a nuclear spin. Their experiment involved probing the interaction of a NV spin with, on average, four carbon-13 atoms, about 1 nm away. The carbon-13 spins – which serve as the environment – are too weak to interact with one another but nevertheless cause decoherence in the NV spin. This process involves the carbon-13 spins changing to new quantum states that depend on the state of the NV spin.
The experiment is done by shining a green laser light onto NV spins within a millimetre-sized sample of diamond and measuring the photons emitted as microwave and radiofrequency fields are switched on and off. Because they were not able to observe the carbon-13 spins directly, the team transferred these spin states to the NV spins and again exploited fluorescence measurements. This counter-intuitive approach was possible, says Jelezko, because the three steps in the experiment – preparing the spin states, decoherence and measurement – were cleanly separated in time.
Natural environment
Doing so, the researchers found the predicted redundancy. By measuring the spin of just one carbon-13 nucleus, and repeating the experiment many times, they found they could correctly deduce most of the NV spin properties most of the time. But measurements of additional nuclear spins added little to this knowledge. These results, they write in a paper accepted for publication in Physical Review Letters and posted on arXiv, “give the first laboratory demonstration of quantum Darwinism in action in a natural environment”.
The term “natural”, says Jelezko, refers to the fact that spin decoherence in solids usually takes place as a result of magnetic interactions with nuclear spins. He adds that this process makes it difficult to build quantum computers using spins in solids. The group’s next step is to scale its experiment up, although Jelezko admits that getting anywhere near the size of a macroscopic object – even a dust particle – is likely to prove impossible. “I don’t think we will be able to do a billion atoms,” he says, “but 20 would already make a big difference”.

Is photosynthesis quantum-ish?
Two other groups, meanwhile, one in Italy and the other in China, have carried out similar measurements (using the polarization of photons) that also show redundancy. These systems are less natural than the nitrogen vacancies but are easier to control, according to one member of the latter group, Chao-Yang Lu of the University of Science and Technology of China in Hefei. As such, Lu says that he and his colleagues were able to demonstrate not only the proliferation of classical information but also an “uptick” in information taking place at the quantum level – something that experimental noise prevents when using nitrogen vacancies.
However, quantum Darwinism is not the only game in town. Adán Cabello, a theoretical physicist at the University of Seville in Spain, argues that the latest experiments “offer only schematic versions of what a real environment consists of,” and that other approaches can reveal crucial insights into the emergence of classical reality. For example, he says, he and colleagues at the University of Stockholm have shown how to make measurements on trapped ions while still preserving some of the system’s quantum coherence. This, he says, shows that measurement “is not an abrupt transition but rather the result of a dynamical process governed itself by quantum mechanics”.